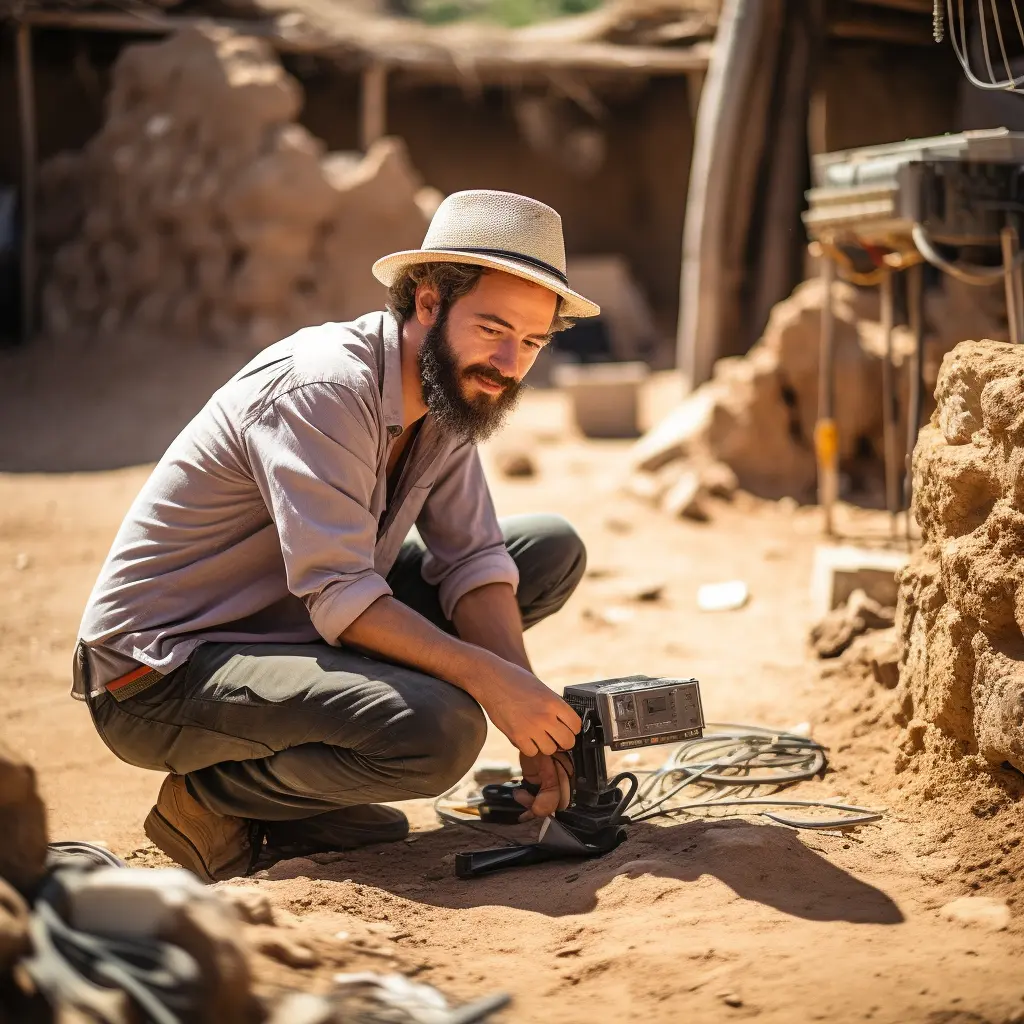
Introduction
Unveiling the tapestry of our past requires a marriage of both art and science. But what happens when two seemingly distinct disciplines, archaeology and physics, intertwine? In this exploration, we aim to showcase the harmony between these fields, drawing from the depths of quantum theories and the vast landscapes of ancient civilizations. As you journey through this piece, be prepared to witness the fusion of knowledge that showcases the true essence of interdisciplinary research.
Brief Overview of Archaeology and Physics
Archaeology, at its core, is the study of human history and prehistory through the excavation of sites and the analysis of artifacts and other physical remains. From the grand pyramids of Giza to the intricate pottery of the Indus Valley, archaeology offers us a window into civilizations that once thrived, their cultures, beliefs, and technological advancements.
On the other hand, physics is a branch of science concerned with the nature and properties of matter and energy. It dives deep into the fundamental forces of the universe, from the behavior of atoms and subatomic particles to the vastness of galaxies. The language of physics is mathematics, and through its lens, we’ve been able to grasp the complexities of our universe, from the tiniest quantum particles to the vast interstellar entities.
Convergence of Two Sciences
One might ponder, how does the realm of electrons, neutrons, and quantum mechanics mesh with the excavation of ancient relics and structures? The answer lies in the tools and techniques that are birthed from this union.
Radiocarbon dating, for instance, a jewel of this convergence, employs the principles of radioactive decay (a physics concept) to date organic materials discovered at archaeological sites. Ground-penetrating radar, another marvel, uses electromagnetic radiation (again, a physics domain) to visualize what lies beneath our feet, potentially unearthing buried artifacts or structures without a single shovel’s thrust into the earth.
This integration not only elevates the precision and depth of archaeological findings but also enriches physics by providing real-world, tangible applications for its theories and principles. The fusion of archaeology and physics is not just a testament to the versatility of science, but a model for how collaborative efforts can yield results greater than the sum of their parts.
Historical Context
Understanding the intricacies of our past often mandates a panoramic view of not just the artifacts and their stories but also the very methods that brought them to light. The union of archaeology and physics is not a sudden twist in the narrative of science but a gradual and deliberate fusion, rooted in the annals of history. Let’s delve deeper into this historical journey.
The Evolution of Archaeological Methods
In its infancy, archaeology was markedly different from the structured and systematic discipline we recognize today. Early excavations were often treasure hunts, led by enthusiasts and adventurers more than by trained scholars. Without standardized techniques, much of the contextual information about discovered items was lost.
As the 19th century dawned, the discipline began to see a seismic shift. Emphasis started leaning towards meticulous documentation, site preservation, and stratigraphic analysis. Stratigraphy, the study of rock layers and layering, offered archaeologists a way to understand the chronology of sites. It was a precursor to understanding that the deeper one digs, the older the artifacts one would find, paving the way for more chronological accuracy.
But even with these advancements, a certain depth of understanding remained elusive. Absolute dating methods were the missing puzzle piece, a void that physics was destined to fill.
The Introduction of Physics in Archaeological Research
Enter the 20th century, and with it, the burgeoning realm of atomic and nuclear physics. Scientists began to understand radioactive decay and its predictable patterns. The most significant boon to archaeology from this revelation was radiocarbon dating. Developed in the late 1940s by physicist Willard Libby, this method used the decay rate of the carbon-14 isotope to accurately date organic materials, providing a time window for artifacts that was previously impossible.
It wasn’t just dating, though. Techniques like X-ray fluorescence allowed for elemental analysis of artifacts without damaging them. Ground-penetrating radar provided non-invasive means to survey and map archaeological sites. And thermoluminescence dating helped in ascertaining the age of ancient pottery.
The inclusion of physics in archaeological research was transformative. It provided tools and methods that not only filled gaps in understanding but also pushed the boundaries of what was deemed knowable. This interplay between the macro (the vast landscapes of archaeological sites) and the micro (the atomic and subatomic worlds of physics) has forever altered the trajectory of historical and prehistorical research.
Radiocarbon Dating (Carbon-14 Dating)
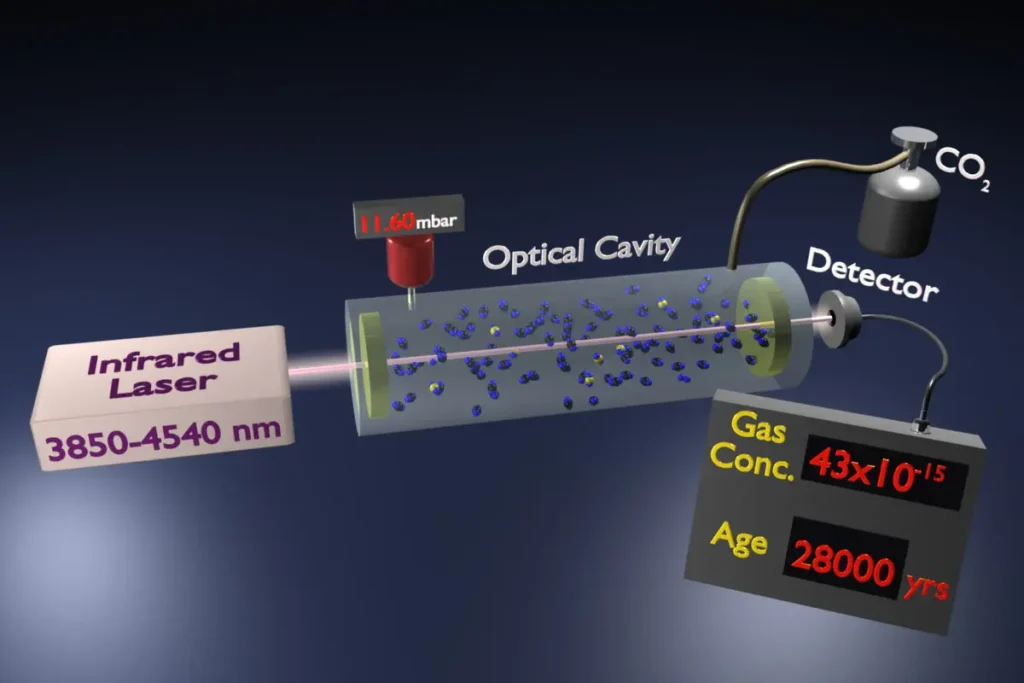
Radiocarbon dating, also known as carbon-14 dating, is an exemplar of how the microscopic nuances of physics can unravel macroscopic histories etched over millennia. This technique, a confluence of archaeology and nuclear physics, has become a cornerstone in determining the age of ancient artifacts. As we delve deeper into this method, the elegance of carbon-14 decay and its paramount importance in dating historical relics becomes apparent.
Principles Behind Carbon-14 Decay
At the heart of radiocarbon dating lies an isotope of carbon known as Carbon-14 (^14C). Unlike the more common Carbon-12 (^12C), which is stable, ^14C is radioactive, and over time, it naturally decays back into nitrogen-14 (^14N) at a predictable rate. This rate of decay is known as its half-life, which for Carbon-14 is approximately 5,730 years.
Here’s the fascinating bit: while a living organism is alive, it constantly absorbs carbon, including both ^12C and ^14C, from the atmosphere. This uptake maintains a steady and known ratio of ^12C to ^14C in the organism. However, upon death, this absorption stops. From this point onward, the ^14C in the deceased organism starts to decay without any new ^14C being added. Over time, the ratio of ^14C to ^12C decreases. By measuring this current ratio in a sample and comparing it to the initial ‘living’ ratio, scientists can determine how many half-lives of ^14C have passed since the organism’s death, thereby estimating its age.
Application in Dating Ancient Artifacts
Given the predictable decay of ^14C, radiocarbon dating has proven invaluable in dating organic artifacts – materials that were once part of a living organism. This includes items like wood, bone, shell, and even some textiles.
Imagine unearthing a wooden tool from an archaeological dig. By analyzing a small sample of this tool, researchers can determine its ^14C to ^12C ratio. Using this data, along with the known half-life of ^14C, they can deduce when the tree from which the tool was made had been felled.
The implications are profound. Radiocarbon dating has been instrumental in dating critical historical events, such as confirming the chronological timelines of ancient Egypt, or clarifying the timelines of the Neolithic period. Additionally, it has assisted in debunking historical forgeries and verifying the authenticity of priceless artifacts.
While radiocarbon dating is a potent tool, it’s also essential to understand its limitations. The technique is most effective for dating artifacts up to about 50,000 years old. Beyond this, the remaining ^14C is so minimal that it becomes challenging to measure accurately.
Ground-Penetrating Radar (GPR)
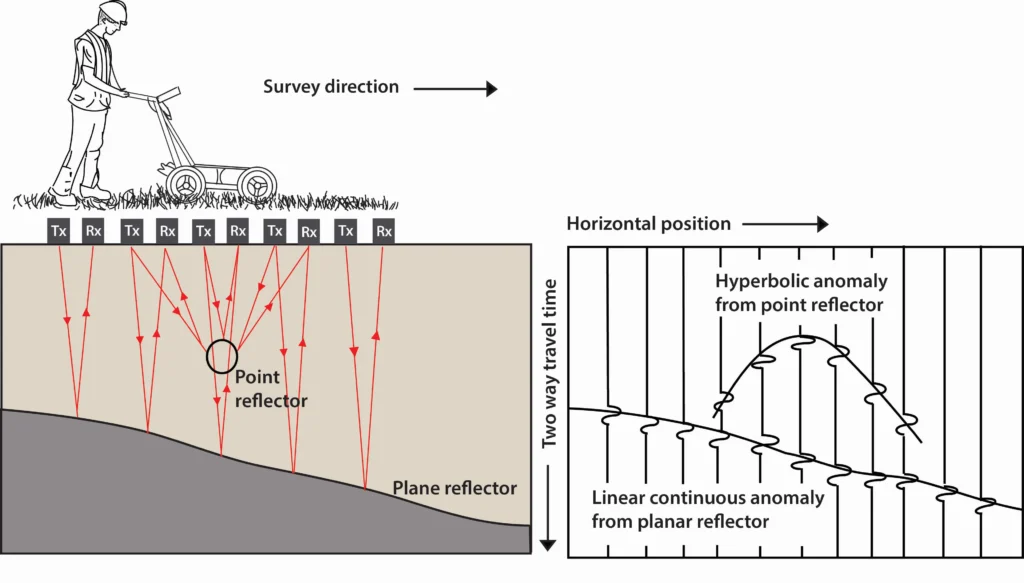
As our quest to uncover the mysteries of the past continues, the tools at our disposal evolve. One such marvel of modern science aiding archaeologists is the Ground-Penetrating Radar, or GPR. This non-invasive geophysical method allows experts to peer beneath the earth’s surface, detecting buried artifacts, structures, and other subsurface anomalies without disturbing the ground. Dive with us into the world of GPR as we explore its mechanics and marvel at its practical applications in the field of archaeology.
Understanding the Mechanics of GPR
GPR, much like the radar systems used in aviation or meteorology, works by emitting electromagnetic waves and then detecting the signals that are reflected back. Here’s a breakdown of how it operates:
- Emission of Waves: A GPR system consists of a transmitting antenna that sends out short pulses of high-frequency electromagnetic waves into the ground.
- Reflection and Detection: As these waves encounter different materials or objects beneath the surface, some get reflected back. The degree to which they’re reflected is determined by the electromagnetic properties of the encountered materials. Materials with contrasting properties (e.g., a stone wall in a soil matrix) will cause stronger reflections.
- Time-Delay Measurement: The GPR measures the time it takes for the waves to be reflected back to the surface. Given the speed of the emitted waves, this time delay can be translated into depth, providing a measure of how deep the object or anomaly is located.
- Creation of an Image: With sophisticated software, the data captured by GPR is processed and visualized, often in the form of a cross-sectional ‘slice’ through the ground. This can reveal buried walls, foundations, graves, and even voids or underground cavities.
Real-world Case Studies of GPR in Excavations
GPR has illuminated many archaeological sites with its profound insights. Here are a couple of noteworthy instances:
- Stonehenge, England: One of the most iconic prehistoric monuments, Stonehenge’s surroundings were meticulously surveyed using GPR. The results were astonishing. A plethora of new sites, including burial mounds and massive prehistoric pits, were discovered, painting a more intricate picture of Neolithic and Bronze Age landscapes.
- Ancient Roman City, Falerii Novi, Italy: GPR enabled archaeologists to map an entire Roman city, Falerii Novi, located 50km north of Rome. Without any excavation, they unveiled a complex network of water pipes, public monuments, and even a market. It showcased the immense potential of GPR in creating a detailed layout of ancient settlements.
These examples underscore the power and precision of GPR in archaeological endeavors. As technology advances, we can anticipate even more profound discoveries, with GPR acting as a bridge between the seen and the unseen, the known and the mysterious.
Thermoluminescence Dating
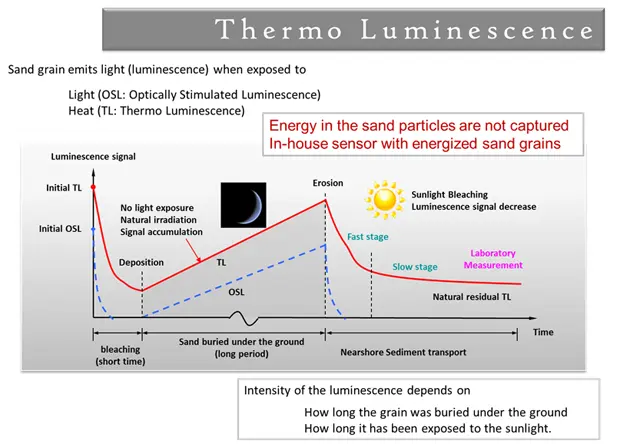
When we gaze upon an ancient ceramic artifact in a museum, seldom do we ponder over its exact age or the methods used to ascertain it. Delving into the world of archaeology, one finds a fascinating intersection where physics meets history. Thermoluminescence dating stands out as a shining example of this confluence. Used predominantly to date ancient pottery and ceramics, this method hinges on the principles of atomic physics to unveil historical timelines. Let’s explore the captivating science behind thermoluminescence and its indispensable role in archaeological dating.
The Science Behind Thermoluminescence
Thermoluminescence (TL) is a phenomenon where certain minerals emit light when heated. This glow results from the release of stored energy in the form of trapped electrons. The foundational science of TL dating revolves around this principle. Here’s a simplified breakdown:
- Electron Trapping: When pottery is fired in its initial creation process, it’s heated to high temperatures. This heating event effectively ‘resets’ the piece, expelling any previously trapped electrons from defects in the mineral’s crystal lattice.
- Exposure to Radiation: Over time, as the pottery is buried, it gets continually bombarded by natural ionizing radiation from its environment, primarily from radioactive elements in the soil and cosmic rays. This radiation excites electrons in the mineral, some of which get trapped in these defects.
- Accumulation of Trapped Electrons: The longer the pottery remains buried, the more electrons get trapped, accumulating like a “chronological clock.”
- Releasing the Stored Energy: When an archaeologist unearths the pottery and wants to date it, a sample is heated in a laboratory. This heating releases the trapped electrons, producing a light or ‘luminescence.’ The intensity of this light is proportional to the number of trapped electrons, and hence, the time that has passed since the pottery was last fired.
Its Role in Dating Ancient Pottery and Ceramics
Thermoluminescence dating has emerged as an invaluable tool, especially when other dating methods like radiocarbon dating are not applicable. Here are its significant contributions:
- Broad Time Range: TL dating is versatile. It can date pottery from a few decades old to several hundreds of thousands of years, providing archaeologists with a broad chronological canvas.
- Authenticity Verification: The art market, unfortunately, sees its fair share of forgeries. TL dating has become a means to verify the authenticity of ancient ceramics. Modern replicas won’t show the same thermoluminescence characteristics as genuine ancient artifacts.
- Complementary Tool: In sites where multiple dating methods are employed, TL acts as a complementary tool, refining the age estimates and adding layers of verification.
- Global Application: From the ancient ceramic relics of East Asia to the prehistoric settlements of Europe, TL dating has had a global impact, enabling scholars to piece together timelines across different cultures and epochs.
The magic of thermoluminescence dating lies in its ability to breathe life into inanimate objects, transforming them from mere relics to vibrant storytellers of our shared past.
Other Physics-Based Techniques in Archaeology
The world of archaeology has embraced modern science in fascinating ways, pushing boundaries and making ground-breaking discoveries that wouldn’t have been possible with traditional methods alone. As we’ve journeyed through various techniques like thermoluminescence and ground-penetrating radar, it’s evident that physics plays a pivotal role in decoding history. Here, we’ll delve into three more advanced techniques: Portable X-ray Fluorescence (pXRF), Magnetic Resonance Imaging (MRI), and Neutron Activation Analysis, and witness how they’re making waves in archaeological research.
Portable X-ray Fluorescence (pXRF)
A relatively new entrant in the archaeological toolkit, portable X-ray fluorescence offers a non-destructive means to analyze materials, primarily for elemental composition. Here’s how it’s making an impact:
- How it Works: When a material is subjected to X-rays, it emits secondary X-rays in response, unique to each element present. By measuring the energy and intensity of these emitted X-rays, pXRF can determine which elements are present and in what quantities.
- Applications:
- Provenance Determination: By understanding the elemental makeup of artifacts, researchers can trace the source of raw materials, like the origin of obsidian in tools.
- Art Authentication: pXRF can discern between authentic ancient pigments and modern forgeries in artwork.
- Understanding Ancient Technologies: Analyzing residues on tools can provide insights into their usage and the materials they processed.
Magnetic Resonance Imaging (MRI) and Archaeology
While MRI is more commonly associated with medical imaging, its applications in archaeology are steadily gaining traction.
- How it Works: MRI leverages the magnetic properties of atomic nuclei, especially hydrogen, to create detailed images of the internal structures of objects without cutting or damaging them.
- Applications:
- Mummies and Burials: MRI can examine mummified remains, revealing information about diseases, injuries, and mummification methods.
- Archaeological Conservation: Before restoring or conserving delicate artifacts, MRI can provide insights into their internal structures, guiding conservation strategies.
Neutron Activation Analysis
A potent tool for elemental analysis, neutron activation analysis (NAA) is prized for its precision and ability to detect even trace elements.
- How it Works: Samples are bombarded with neutrons, making the elements within them radioactive. As these radioactive elements decay, they emit gamma rays, which can be measured to determine the type and quantity of elements present.
- Applications:
- Clay Source Identification: By analyzing pottery’s elemental composition, researchers can pinpoint where its clay was sourced, shedding light on ancient trade routes and cultural interactions.
- Food Residue Analysis: Identifying trace elements can help infer the types of food once stored in pottery or cooked on ancient hearths.
Conclusion
As our expedition into the heart of archaeology and physics comes to a close, we are left with a profound appreciation for the depths of knowledge these fields offer when combined. The relationship between physics and archaeology isn’t merely transactional; it is transformative. Both realms, with their distinct languages of the universe, come together to narrate stories of our past in unparalleled clarity. Let’s encapsulate our journey and muse over the horizons that lie ahead.
Recap of the Synergy Between Physics and Archaeology
Physics, with its precise, quantifiable measurements and universal laws, brings a unique lens to the world of archaeology. We’ve seen the incredible:
- Applications of Radiocarbon Dating: Through the predictable decay of carbon-14, we can pinpoint the age of organic remains with remarkable accuracy.
- Advancements with Ground-Penetrating Radar: Revealing hidden structures and buried artifacts without even moving a shovel’s worth of dirt.
- Magic of Thermoluminescence: Lighting up the historical timelines of ancient pottery.
- Insights from Techniques like pXRF, MRI, and NAA: These tools, each in their own right, have revolutionized the way we analyze, preserve, and understand artifacts.
At every twist and turn, physics has provided archaeology with the tools, methodologies, and perspectives to dig deeper (both figuratively and literally) into our history.
Encouraging Further Exploration and Study
The confluence of archaeology and physics is a testament to the boundless potential of interdisciplinary collaboration. To budding archaeologists, physicists, and curious minds alike:
- Stay Curious: The answers to many archaeological questions lie in the innovative application of physical principles. Stay updated with advancements in both fields.
- Seek Collaboration: The most groundbreaking discoveries often come from diverse teams with varied expertise. Collaboration is the key.
- Educate and Share: Share findings, methodologies, and insights with the broader community. The propagation of knowledge enriches us all.
- Look to the Horizon: As technology advances and our understanding of physics expands, so too will our archaeological toolkit. There are still countless mysteries buried beneath our feet, waiting to be unearthed.
In the dance of electrons, protons, and photons, we find the rhythm of civilizations past. Here’s to many more discoveries, insights, and stories waiting to be told.